Importance Score: 35 / 100 🔵
Scientists glean much of their understanding of the early solar system from meteorites – ancient space rocks that traverse interplanetary space and withstand a fiery descent through Earth’s atmosphere. Among these meteorites, a specific type known as carbonaceous chondrites is considered the most primitive, offering a unique window into the solar system’s nascent stages. These carbonaceous meteorites are crucial for studying the origins of our planetary neighborhood.
Composition and Significance of Carbonaceous Chondrites
Carbonaceous chondrites are abundant in water, carbon, and organic compounds. They are classified as “hydrated,” indicating the presence of water molecules chemically bound within the minerals that constitute the rock. The water’s components are integrated into the crystalline structures. Many researchers propose that these ancient space rocks played a vital role in delivering water to the early Earth.
Prior to encountering Earth, space rocks are generally categorized as asteroids, meteoroids, or comets, based on their size and composition. Should a fragment of such an object successfully reach Earth’s surface, it is then designated a “meteorite.”
Astronomical observations of asteroids reveal that a majority possess water-rich, carbonaceous compositions. Theoretical models suggest that over half of all meteorites should similarly be carbonaceous. However, less than 4% of the meteorites discovered on Earth are of the carbonaceous variety. This significant discrepancy raises the question: why the imbalance?
A recent study, detailed in the journal Nature Astronomy on April 14, 2025, undertaken by planetary scientists, including myself, sought to address this longstanding question: What accounts for the apparent scarcity of carbonaceous chondrites among meteorite finds?
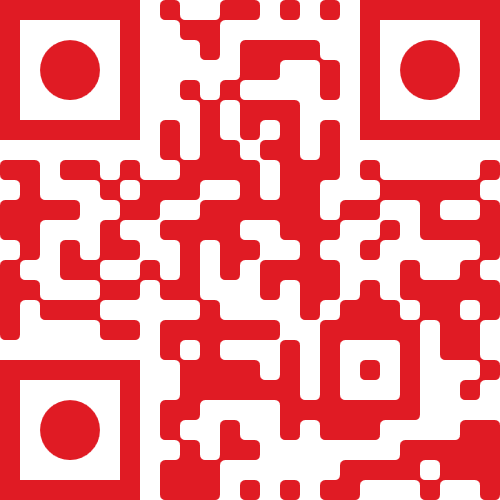
vCard.red is a free platform for creating a mobile-friendly digital business cards. You can easily create a vCard and generate a QR code for it, allowing others to scan and save your contact details instantly.
The platform allows you to display contact information, social media links, services, and products all in one shareable link. Optional features include appointment scheduling, WhatsApp-based storefronts, media galleries, and custom design options.
Unlocking Secrets Through Sample-Return Missions
The scientific community’s eagerness to examine these primordial space rocks has spurred recent sample-return space exploration missions. NASA’s OSIRIS-REx and JAXA’s Hayabusa2 missions have revolutionized our understanding of primitive, carbon-rich asteroids. These missions are critical in deciphering the composition of early solar system bodies.
Meteorites found on Earth’s surface are subject to terrestrial weathering from rain, snow, and biological activity, potentially altering their original state and complicating analysis. To circumvent this, the OSIRIS-REx mission was launched to asteroid Bennu to collect an uncontaminated sample. Retrieving this pristine material allowed scientists to conduct detailed investigations into the asteroid’s intrinsic makeup.
Similarly, Hayabusa2’s expedition to asteroid Ryugu secured pristine samples from another asteroid with a comparable water-rich composition. These missions provide invaluable samples for in-depth study.
Collectively, these missions have enabled planetary scientists to directly analyze unaltered, delicate carbonaceous material from asteroids. These asteroids serve as a direct portal to the fundamental constituents of our solar system and the origins of life itself. Studying these samples is key to understanding our cosmic origins.
Addressing the Carbonaceous Chondrite Paradox
For many years, scientists operated under the assumption that Earth’s atmosphere acted as a filter, selectively excluding carbonaceous cosmic debris.
When a celestial object enters Earth’s atmosphere, it endures significant pressure and intense heat. Carbonaceous chondrites are characteristically more fragile and crumbly compared to other meteorite types, reducing their likelihood of atmospheric survival. Their structural weakness makes them vulnerable during atmospheric entry.
Meteorites commonly originate from collisions between asteroids, events that generate numerous rock fragments ranging from centimeters to meters in size. These cosmic fragments traverse the solar system and can eventually descend to Earth. Objects smaller than a meter are classified as meteoroids.
Meteoroids are generally too small for telescopic detection, except in rare instances when they are on a collision course with Earth, and astronomers happen to observe them. Observing these small objects directly is challenging.
However, an alternative approach exists for scientists to study this population and gain insights into the compositional disparities among meteorites. Utilizing Earth’s atmosphere as a detection tool offers a novel perspective.
Leveraging Meteor and Fireball Observation Networks
Our research team employed Earth’s atmosphere as a natural observatory.
While the majority of meteoroids entering Earth’s atmosphere are minute, sand-sized particles, larger bodies, up to a couple of meters in diameter, occasionally impact. Estimates suggest that approximately 5,000 metric tons of micrometeorites reach Earth annually. Furthermore, each year, an estimated 4,000 to 10,000 larger meteorites, roughly golf ball-sized and above, make landfall – equating to more than 20 each day. Earth is constantly bombarded by space debris.
The advent of digital cameras has made continuous, 24/7 monitoring of the night sky both feasible and cost-effective. Affordable, highly sensitive sensors and automated detection software empower researchers to survey extensive portions of the night sky for bright flashes, indicative of a meteoroid entering the atmosphere. Technological advancements have revolutionized meteor observation.
Researchers can analyze these continuous streams of data using automated analysis techniques – or diligent researchers – to extract critical data. Sophisticated algorithms aid in the analysis of meteor events.
Our team operates two global networks: FRIPON, a French-led initiative with stations across 15 nations, and the Global Fireball Observatory, a collaborative effort originating from the Desert Fireball Network in Australia. In conjunction with other publicly accessible datasets, my colleagues and I analyzed the trajectories of nearly 8,000 atmospheric entry events recorded by 19 observation networks spanning 39 countries. Global collaboration strengthens our understanding of meteor impacts.
By contrasting all meteoroid impacts observed entering Earth’s atmosphere with those that successfully survive and are recovered as meteorites, we can determine which asteroids produce fragments robust enough to endure atmospheric passage. Conversely, we can also identify asteroids that yield weaker materials less frequently found as meteorites on Earth. Comparing atmospheric events and meteorite finds is crucial.
Solar Heating as a Decisive Factor
Surprisingly, our findings indicate that a significant number of asteroid fragments fail to reach Earth’s surface. A process appears to eliminate weaker materials while these fragments are still in space. The fragile nature of carbonaceous material suggests it is susceptible to degradation due to thermal stress when its orbit brings it close to the Sun. Space-based processes significantly impact meteorite populations.
As carbonaceous chondrites orbit, experiencing cycles of solar proximity and distance, the resulting temperature variations induce cracks within their structure. This thermal cycling effectively fragments and removes less robust, hydrated boulders from the population of near-Earth objects. Post-thermal cracking, surviving fragments still face the challenge of atmospheric entry. Thermal stress weakens carbonaceous materials in space.
Only a fraction, approximately 30%-50%, of the remaining objects survive the atmospheric transit and become meteorites. The fragments originating from orbits that approach closer to the Sun tend to be considerably more resilient, enhancing their odds of surviving the arduous passage through Earth’s atmosphere. This phenomenon is known as survival bias. Durable materials are more likely to become meteorites.
For decades, scientists have primarily attributed the relative scarcity of carbonaceous meteorites to atmospheric filtering. However, our research suggests that a substantial portion of the removal process occurs earlier, in the vacuum of space. Space-based destruction, not just atmospheric filtering, explains carbonaceous meteorite rarity.
Looking ahead, advancements in scientific methods can further validate these conclusions and improve our ability to identify meteoroid compositions. Enhanced telescopic capabilities to detect objects immediately before Earth impact are needed. More sophisticated modeling of atmospheric breakup dynamics will also aid in studying these objects. Future research will refine our understanding of meteoroid origins.
Finally, future investigations can focus on refining techniques to determine the composition of fireballs based on the colors of meteors, offering remote compositional analysis. Analyzing meteor colors could reveal their composition non-invasively.